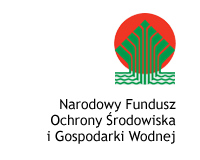
National Fund of Environmental Protection and Water Management on behalf of the Ministry of Environment
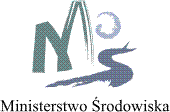
- INTRODUCTION (Piotr Krzywiec, Marek Jarosiński)
- SELECTED PHYSICAL PARAMETERS OF MINERALS AND ROCKS, AND THEIR APPLICATION FOR GEOPHYSICAL DATA INTERPRETATION (Nonna Bakun-Czubarow)
- IMPORTANCE OF VARIATIONS OF GEOMECHANICAL PARAMETERS OF MAGMATIC AND METAMORPHIC ROCKS UNDER HIGH PRESSURE AND TEMPERATURE (Joanna Pinińska)
- SEISMIC MODELS OF THE CRUSTAL STRUCTURE OF THE TRANS-EUROPEAN SUTURE ZONE (TESZ) IN NW POLAND (Marek Grad, Aleksander Guterch)
- LOWER CRUSTAL AND UPPERMOST MANTLE ROCKS WITHIN THE AREA OF THE POLONAISE’97 PROJECT: PETROLOGICAL – SEISMIC MODELS (Jacek Puziewicz)
- THERMAL LITHOSPHERIC MODEL OF THE CENTRAL AND NORTH-WESTERN POLAND (Jacek Majorowicz, Marta Wróblewska)
- INTEGRATED GRAVITY AND MAGNETIC MODELLING ALONG P4 SEISMIC PROFILE (POLONAISE’97 PROJECT) (Zdzisław Petecki)
- STRUCTURE OF THE LITHOSPHERE ALONG THE P2 AND P4 PROFILES (POLONAISE'97 PROJECT) – 2D THERMAL-GRAVITY MODELLING (Olga Polechońska, Marta Wróblewska)
- EDGE OF THE EAST-EUROPEAN CRATON IN THE NORTHWESTERN AND CENTRAL POLAND IN THE LIGHT OF SEISMIC, MAGNETIC AND GEOTHERMAL DATA (Czesław Królikowski)
- MID-POLISH TROUGH BASEMENT TECTONIC STRUCTURE BASED ON ANALYSIS OF SEISMIC REFLECTION, GRAVITY AND MAGNETIC DATA (Piotr Krzywiec, Stanisław Wybraniec, Zdzisław Petecki)
- INTERPRETATION OF DEEP SEISMIC DATA – RESULTS OF SEISMIC REFLECTION MODELLING (Kaja Pietsch, Marcin Kobylarski)
- ONE-DIMENSIONAL RHEOLOGICAL LITHOSPHERIC MODELS ALONG THE POLONAISE’97 PROJECT, TTZ AND LT DEEP SEISMIC PROFILES (Marek Jarosiński, Marcin Dąbrowski)
- GEOLOGICAL INTERPRETATION OF DEEP SEISMIC REFRACTION DATA OF THE POLONAISE’97 PROJECT – PALAEOZOIC PLATFORM (Stanisław Mazur, Marek Jarosiński)
- GEOLOGICAL INTERPRETATION OF DEEP SEISMIC REFRACTION DATA OF THE POLONAISE’97 PROJECT – PRECAMBRIAN PLATFORM (Zbigniew Cymerman)
INTRODUCTION
Piotr Krzywiec, Polish Geological Institute, Warsaw;
Marek Jarosiński, Polish Geological Institute, Warsaw
The study area was defined by location of the deep refraction profiles of the POLONAISE’97 project (fig. 1)
. This seismic database together with other available geophysical data was used for integrated geological – geophysical analysis of the structure of the Earth’s crust and lithosphere. Main research tasks that have been completed within this research project included:
- interpretation and modeling of seismic refraction and reflection data
- interpretation and modeling of gravity data
- interpretation and modeling of magnetic data interpretation and modeling of thermal data
- rheological modelling
- geodynamic analysis
Part of the completed research had partly methodological character (e.g. combined thermal – gravity modelling) and required new research tools to be developed (e.g. rheological modelling). Main task of the project was was most comprehensive integration of geophysical and petrophysical data available within the study area (central and northern Poland). First four research tasks (analysis of seismic, gravity, magnetic and thermal data) were of entirely geophysical character. However, even during this part of the project numerous geological concepts have ben widely analysed and applied. Fifth task (rheological modelling) considerably extende beyond the classical studies and considered widely understood problems of the geomechanics of the lithosphere. Sixth task (geodynamical analysis) focused on various geological aspects of geophysical data interpretation. It must be stressed however, that final comprehensive geological analysis was not the main task of this project. Completed geological – geodynamic analysis was used to show relationship between geophysical and geomechanical results and possible geological interpretations. Full comprehensive geological synthesis will evolve together with newly constructed models of accretion of lithospheric blocks along the edge of the Baltica and their later evolution. Results obtained in the course of this research project have been presented at the following conferences:
- Vth International Meeting „Heat Flow And The Structure Of The Lithosphere” Kostelec nad Čarnym Lessy, Czechy, 10 – 16 czerwca 2001r Abstrakt: J. Majorowicz, M. Wróblewska, J. Szewczyk oraz P. Krzywiec:” Heat flow models across the Trans-European Suture Zone in the area of the POLONAISE’97 international seismic experiment – Polish Lowland.” p.50
- Joint meeting of EUROPROBE TESZ, TIMPEBAR, URALIDES & SW-IBERIA projects "EUROPROBE Neoproterozoic-Early Paleozoic, Time-Slice Symposium: Orogeny and Cratonic Response on the Margins of Baltica", Ankara, Turcja, 2001: J. Majorowicz, M. Wróblewska, J. Szewczyk oraz P. Krzywiec:” Heat flow models across the rans-European Suture Zone in the area of the POLONAISE’97 international seismic experiment.”, p.44.
- Majorowicz J., Cermak V., Safanda J., Wróblewska M., Krzywiec P.,Grad M., Guterch A., 2002, Geophysical consequences of the craton-accreted terranes thermal transition in Poland. 27th General Assambly, European Geophysical Society, Nice, France, 21-26.04.2002, abstract EGS02-A-03439.
- Grad M., Guterch A., Jarosiński M., Królikowski C, Krzywiec P., Majorowicz J., Petecki Z., Wróblewska M., Wybraniec S., 2003, Integrated geophysical – rheological model of the Trans-European Suture Zone (TESZ) in NW Poland. European Geophysical Society Geophysical Research Abstracts, 5, 01725.
- Majorowicz J.A., Čermak V., Šafanda J., Krzywiec P., Wróblewska M., Guterch A., Grad M., 2003, Heat flow models of the transition zone between Palaeozoic and Precambrian Europe based on Polonaise’97 deep seismic data. [w]: Goleby B., Drummond B., Bannister S., Henrys S., (comp.), The 10th International Symposium on Deep Seismic Profiling of the Continents and their Margins, Programme and Abstracts, Taupo, New Zealand, 6-10.01. Institute of Geological and Nuclear Sciences Information Series, 52.
Results obtained in the course of this research project have been presented in the following publications:
- Jarosiński M., Poprawa P., Dąbrowski M., 2002. 1D modelling of Lithosphere’s rheology – an overview of methodology. Przegląd Geologiczny, 50(10/1): 879-892 (in Polish with English abstract).
- Majorowicz J., Wróblewska M., Krzywiec P., 2002, Interpretation and modelling of Earth’s heat flow within the area of POLONAISE’97 seismic experiment – critical analysis. Przegląd Geologiczny, 50(11): 1082-1091 (in Polish with English abstract).
- Majorowicz J.A., Cermak V., Safanda J., Krzywiec P., Wróblewska M., Guterch A., Grad M.,2003. Heat flow models across the Trans-European Suture Zone in the area of the POLONAISE’97 seismic experiment. Physics and Chemistry of Earth, 28: 375 – 391 ( pdf [pdf.] (870 KB) )
- Majorowicz J., 2004. Thermal lithosphere across the Trans-European Suture Zone in Poland. Geological Quarterly, 48(1): 1-14 ( pdf [pdf.] (962 KB) )
1. SELECTED PHYSICAL PARAMETERS OF MINERALS AND ROCKS, AND THEIR APPLICATION FOR GEOPHYSICAL DATA INTERPRETATION
Nonna Bakun-Czubarow, Institute of Geological Sciences, Polish Academy of Sciences, Warsaw
The aim of the paper was to collect the select physical parameters (ρ,Vp, Vs) of major minerals and lithospheric rocks from all over the world, as well as to present their changes as functions of pressure and temperature. The physical parameters were presented in four tables. In the first table, there are given densities and P wave velocities as a function of P in the range of 0.1 – 10.0 kbar for 137 crustal and lower lithospheric rocks. In the second table the densities and Vp velocities under pressure ranging from 0.1 to 30 kbar, for ultramafic components of lower lithosphere are presented (fig. 2)
. The third table contains velocities of P and S waves in chosen igneous, sedimentary, and metamorphic rocks as function of temperature (25–300 oC)and pressure (0.5-6.0 kbar). Within the given limits, temperature and pressure seem to have opposite influence on the discussed physical parameters of the rocks. The fourth table is devoted to the Vp anisotropy of monocrystals of major minerals. There are also given mean values of densities as well as P and S wave velocities for these minerals. In petrological modelling of Earth’s crust and mantle, the concordance with tectonics, geology and rocks available in the area, as well as the nature of seismic discontinuities should be considered. For example, on the (fig. 3) the idea that Moho discontinuity has the nature of gabbro→eclogite transformation is postulated. Moreover, the position of phase separation boundaries strongly depends upon chemical composition of the rocks.
2. IMPORTANCE OF VARIATIONS OF GEOMECHANICAL PARAMETERS OF MAGMATIC AND METAMORPHIC ROCKS UNDER HIGH PRESSURE AND TEMPERATURE
Joanna Pinińska, Department of Geology, Warsaw University, Warsaw
Geological identity of deep seated structures, not accessible for direct observation relates can be derived from the interpretation of seismic and gravimetric data. On the other hand, the geophysical raw data are interpreted on the basis of extrapolated geological field- and laboratory data. Thus, the positive feed-back exists between the geological and geophysical surveys. With regard to the determination of change of geomechanical parameters of rocks mass under extreme pressures and temperature, special experiments are carried out. Especially, the correlation changes between a rock density and an acoustic wave velocity is determined under increased pressure and temperatures. Resulting curve is being extrapolated, giving an outline of suspect properties of rocks under extreme conditions not subjective to direct investigations. The diagnostic key can be just established for recognition of rock media on the base of their remote sensing by seismic and gravimetric surveys. Geophysical data interpretation is then verifiable by assumed petrophysical parameters established in geomechanical laboratories for certain rock media, and the geological assumptions are being verified by geophysical data. A final identity of the rock mass can be established after detail iterative investigations. For the rocks available close to the Earth surface it is an easy task, and a lot of data are already available. For the deeper zone of lithosphere, the available data are scarce. In the laboratory, experimental determination of rock properties under high temperature and pressure is difficult and costly, because high pressure chambers with heaters, which simulate deep crust conditions are very expensive. For that reason, only selected laboratories financed by petroleum industry, atomic energy institutes and toxic waste projects can achieve technical means enough for determining changes of wave velocity and a rock density at depth. Within the crust in the near surface zone in Poland non-weathered and non-fractured rocks are characterized by longitudinal ultrasonic wave velocity ranging 1400 to 8000 m/s, while volume density varies from about 1,5 g/cm3 do ponad 3 g/cm3 (fig. 4). According to magmatic and metamorphic rock properties data selected from various places worldwide (R. S. Carmichael; Physical Properties of Rocks and Minerals ed. CRC Press 1990) as well as in Poland (Pinińska J., Właściwości wytrzymałościowe i odkształceniowe skał. tom 1 do 8. wyd. UW, 1994 do 2004), the characteristic rock parameters important in seismic and gravimetric studies, are variable under varying conditions of temperature, pressure and pore water contents. E.g. extreme velocities of longitudinal wave propagation (Vp) = 8100 m/s as determined for eclogit and dunite increases in the presence of pore water to 8400 m/s, and (according to R. S. Carmichael data) at the 200 oC and under 500 Mpa pressure reaches up to 8900 m/s (fig. 4). while the transversal wave propagation (Vs) reaches 4510 m/s at 200oC and the 500 Mpa pressure and decreasing at 300 oC to 4440 m/s at dunit. The extreme values of volume density reached in dunit under 500 Mpa laboratory pressure at 200 oC increases from 3.32 g/cm3 to 3.47 g/cm3.
3. SEISMIC MODELS OF THE CRUSTAL STRUCTURE OF THE TRANS-EUROPEAN SUTURE ZONE (TESZ) IN NW POLAND
Marek Grad, Institute of Geophysics, Warsaw University, Warsaw
Aleksander Guterch, Institute of Geophysics, Polish Academy of Sciences, Warsaw
All of the crustal models in SW Poland show generally the same strong structural variations from the Palaeozoic Platform in the southwest, across the TESZ region, onto the EEC to the northeast. The Polish basin is a large structure that filled with sedimentary strata during the Palaeozoic and Mesozoic. The fill in this basin (Vp< 6.0 km/s) reaches thicknesses of ~20 km. This basin is asymmetric, with its northeast margin being most abrupt. The crystalline crust under this basin in only ~ 20 km thick today, indicating that the lithosphere of Baltica was either thinned drastically or terminated along the northeast margin of the basin. The crust of the accreted terranes to the southwest is relatively thin and similar to that found in other noncratonal areas of Western Europe. The East European craton has thick (~ 45 km) three-layered crust. The lower crust is relatively fast (vp> 7.0 km/s), however, lower values to the southwest may indicate the termination of Baltica. High velocity (~ 8.35 km/s) uppermost mantle lies beneath the Avalonia/Variscan terranes and may be due to rifting and/or subduction. A seismic reflector generally occurs at about 10 km depth below Moho throughout the study area and adjacent areas, independent of the actual depth to the Moho. In general, the reflectivity of the uppermost mantle. (Fig. 5)
References:
CZUBA W., GRAD M., LUOSTO U., MOTUZA G., NASEDKIN V., POLONAISE P5 WORKING GROUP (2001) Crustal structure of the East European Craton along POLONAISE'97 P5 profile. Acta Geophys. Pol., 49 (2): 145-168.
CZUBA W., GRAD M., LUOSTO U., MOTUZA G., NASEDKIN V., POLONAISE P5 WORKING GROUP (2002) Upper crustal seismic structure of the Mazury complex and Mazowsze massif within East European Craton in NE Poland. Tectonophysics, 360: 115-128.
GRAD M., JANIK T., YLINIEMI J., GUTERCH A., LUOSTO U., TIIRA T., KOMMINAHO K., ŚRODA P., HÖING K., MAKRIS J., LUND C.-E. (1999) Crustal structure of the Mid-Polish Trough beneath the Teisseyre-Tormquist Zone seismic profile. Tectonophysics, 314: 145-160.
GRAD M., KELLER G.R., THYBO H., GUTERCH A., POLONAISE WORKING GROUP (2002a) Lower lithospheric structure beneath the Trans-European Suture Zone from POLONAISE'97 seismic profiles. Tectonophysics, 360: 153-168.
GRAD M., GUTERCH A. MAZUR S. (2002b) Seismic refraction evidence for crustal structure in the central part of the Trans-European Suture Zone in Poland. Geological Society, London, Special Publications, 201: 295-309.
GRAD M., ET AL. (2003) Crustal structure of the Trans-European suture zone region along POLONAISE’97 seismic profile P4. J. Geophys. Res., 108 (B11): 2541, doi:10.1029/2003JB002426.
GUTERCH A., GRAD M., MATERZOK R., PERCHUĆ E. (1986) Deep structure of the Earth’s crust in the contact zone of the Palaeozoic and Precambrian platforms in Poland (Tornquist-Teisseyre Zone). Tectonophysics, 128: 251-279.
GUTERCH A., GRAD M., JANIK T., MATERZOK R., LUOSTO U., YLINIEMI J., LÜCK E., SCHULZE A., FÖRSTE K. (1994) Crustal structure of the transitional zone between Precambrian and Variscan Europe from new seismic data along LT-7 profile (NW Poland and eastern Germany). C. R. Acad. Sci. Paris, 319, serie II: 1489-1496.
GUTERCH A., GRAD M., THYBO H., KELLER G. R., THE POLONAISE WORKING GROUP (1999) POLONAISE’97 - an international seismic experiment between Precambrian and Variscan Europe in Poland. Tectonophysics, 314: 101-121.
JANIK T., YLINIEMI J., GRAD M., THYBO H., TIIRA T., POLONAISE P2 WORKING GROUP (2002) Crustal structure across the TESZ along POLONAISE'97 seismic profile P2 in NW Poland. Tectonophysics, 360: 129-152.
JENSEN S. L., JANIK T., THYBO H., POLONAISE PROFILE P1 WORKING GROUP (1999) Seismic structure of the Palaeozoic Platform along POLONAISE’97 profile P1 in northwestern Poland. Tectonophysics, 314: 123-143.
ŚRODA P., POLONAISE PROFILE P3 WORKING GROUP (1999) P- and S-wave velocity model of the southwestern margin of the Precambrian East European Craton; POLONAISE'97, profile P3. Tectonophysics, 314: 175-192.
ŚRODA P., CZUBA W., GRAD M., GUTERCH A., GACZYŃSKI E., POLONAISE WORKING GROUP (2002). Three-dimensional seismic modelling of crustal structure in the TESZ region based on POLONAISE'97 data. Tectonophysics, 360: 169-185.
WILDE-PIÓRKO M., GRAD M., TOR WORKING GROUP (2002). Crustal structure variation from the Precambrian to Palaeozoic platforms in Europe imaged by the inversion of teleseismic receiver functions - project TOR. Geophys. J. Int., 150: 261-270.
4. LOWER CRUSTAL AND UPPERMOST MANTLE ROCKS WITHIN THE AREA OF THE POLONAISE’97 PROJECT: PETROLOGICAL – SEISMIC MODELS
Jacek Puziewicz, Institute of Geological Sciences, Wrocław University, Wrocław
Mineral composition of middle and lower crust in Polish part of the Trans-European Suture Zone and neighbouring areas was estimated combining seismic data, geological evolution of the area and exposed cross sections of middle- to lower crust elsewhere in the world (fig. 6). Lower crust in parts of the East European Craton adjoining TESZ is dominated by mafic granulites, with the amount of mafic minerals and/or their Mg/(Mg+Fe) ratio increase towards SW. The overlying parts of middle crust are significantly richer in feldspars and quartz. The amount of those components increases towards the SW, supposedly due to granitic/charnockitic intrusives accumulated at the margin of the EEC. The middle and lower crust of the TESZ is supposedly a prolongation of those from the EEC, thinned and with potentially more intense foliaton. It is overlied by a pile of low-grade metamorphic rocks of the Avalonian accretionary prism. The lower crust of the located to the SW Variscan platform is supposedly the layered one similar to that exposed in the Ivrea Zone, overlied of thick middle crust formed at a relatively hot conditions and injected with numerous granitic plutons.
5. THERMAL LITHOSPHERIC MODEL OF THE CENTRAL AND NORTH-WESTERN POLAND
Jacek Majorowicz, Northern Geothermal, Edmonton, Canada oraz University of North Dakota, Northern Plains Climate Research Center, USA
Marta Wróblewska, Polish Geological Institute,Warsaw
Two dimensional thermal modeling was performed based on the finite difference method of a numerical steady state heat conduction equation, along seismic profiles of the POLONAISE’97 experiment and also TTZ, LT-7, LT-5, LT-4, LT-2. Seismic results were used for constructing the model geometry. In the case of profiles P2 P4 and LT-7 the finite element method was applied for thermal parameter analyzing i.e. thermal conductivity and radiogenic heat production being model variables. As a boundary condition for all models, we have used 8 oC mean annual surface temperature. The preliminary modeling was optimized with surface heat flow (Q) after Karwasiecka and Bruszewska (1997), but the final results use Q values corrected for the paleo- climate effect of the last glaciation (Szewczyk i Gientka, 2003). Heat production was estimated from the statistical relationship with seismic velocity (Rybach and Buntebarth, 1982, 1984). It was corrected for the effects of temperature and pressure after Cermak and Bodri (1986). Crustal heat generation explains only part of the high flow in the zone with thick meta-sediments reaching 20 km. The model of thermal conductivity is based on data published by Chapman and Furlong (1992) and it is explicitly pressure- (depth) and temperature-dependent. Thermal conductivity measured on core samples from the well located in the seismic profiles neighborhood were also used to constrain the conductivity model of the sedimentary fill. The maps of heat flow density, temperature at Moho and thermal lithosphere thickness (taken to be 1300oC) were constructed from the modeling result (Majorowicz et al, 2003 and Majorowicz, 2004). Moho temperature distribution varies from 400 oC to 800 oC within the Polish Lowland area. It is less than 450 oC in SW Poland. The central part of TTZ reaches higher temperatures from 600 oC to 800 oC. The relatively high temperatures at the Moho in the high heat flow area in the south-west Poland are not supported by high Pn seismic velocities at the top of the mantle. High velocities 8.25-8.4 km/s (Jensen et al, 1999 and Guterch and Grad, 2000) suggest a rather cool top of the mantle and low heat flow through the study area for the common mantle petrology. This discrepancy can be partially explained with seismic velocity anisotropy and abnormal compositional variations. Transient character of the lithospheric temperature field where the upper crust still reflects conditions of the high Moho heat flow occurring in the past whereas the lower crust and the upper mantle have already adjusted to the new conditions of the lower Moho heat flow is another explanation (Majorowicz, 2004, Lotz, 2004). However, specification of the process and its timing has not been modeled. Analysis of 2D models proves existence of an extensive temperature increase at the crust-mantle boundary, coherent with the surface heat flow anomaly located at the Dolsk fault region and the Variscan Deformation Front. High heat flow measured in the thin crust area and low heat flow in the thick crust are confirmed by the basic isostasy balance model. Such a model requires that in the area with low elevation (below 200 m) and small morphology variation and high changes of crustal thickness (from 30 to 45 km) high heat flow oscillations are present (adequately 35 to 75 mW/m2). (Fig. 7)
References:
Cermak, V., Bodri, L., 1986. Two-dimensional temperature modeling along five East-European geotraverses. J.Geodynamics, 5, pp.133-163.
Chapman, D.S., Furlong, K.P., 1992. Thermal state of the continental lower crust. In: D.M.Fountain, R.Arculus and R.W.Kay (Eds) Continental lower crust. Elsevier, Amsterdam -London - New York, pp.179-199.
Guterch, A., Grad, M. 2000, New generation of deep lithosphere sounding project: seismic experiments POLONAISE’97 i CELEBRATION 2000 in Central Europe. Prz. Geol., 48: 1085-1095.(in Polish with English abstract)
Jensen, S.L., Janik, T., Thybo, H., POLONAISE WORKING Group, 1999. Seismic structure of the paleozoic platform along POLONAISE '97 profile P1 in north-western Poland. Tectonophys., 314, 123-143.
Karwasiecka M., Bruszewska B., 1997- Heat Flow Density in Poland. CAG PGI, project nr 21/98, Warsaw, (unpublished).
Lotz B., 2004. Neubewertung des rezenten Warmestroms im Nordostdeutschen Becker. GeoForschungsZentrum Potsdam, Scientific Technical Report STR04/04
Majorowicz J., 2004. Thermal lithosphere across the Trans-European Suture Zone in Poland. Geological Quaterly v.48, no.1 : 1-14 ( pdf [pdf.] (962 KB) )
Majorowicz, J.A. Cermak, V., Safanda, J., Krzywiec, P., Wróblewska, M., Guterch, A. And Grad, M., 2003, Heat flow models across the Trans-European Suture Zone in the area of the POLONAISE’97 seismic experiment, Phys. Chem. Earth, 28, 375-391.( pdf [pdf.] (870 KB) )
Rybach, L., Buntebarth, G., 1982. Relationship between the petrophysical properties density, seismic velocity, heat generation and mineralogical constitution. Earth Planet. Sci. Lett. 57, 367-376.
Rybach, L., Buntebarth, G., 1984. The variation of heat generation density and seismic velocity with rock type in the continental crust. Tectonophysics, 103, 309-344. Szewczyk, J. and Gientka D., 2003, Climate and climatic change from underground temperatures: Continental energy balance, land - surface processes, integration with meteorological and proxy data, EGS/AGU 2003 abstract CL19 and poster, EGS/AGU 2003, April, Nice, France.
6. INTEGRATED GRAVITY AND MAGNETIC MODELLING ALONG P4 SEISMIC PROFILE
Zdzisław Petecki, Polish Geological Institute,Warsaw
In order to recognize the lithospheric structure of the contact zone of the Precambrian East European Craton (EEC) and its foreland (Trans-European Suture Zone – TESZ) an integrated gravity and magnetic data modelling has been performed along refraction/wide-angle reflection seismic profile P4. Gravity and magnetic model of the crust and upper mantle down to a depth of 60 km along the P4 profile has shown that geological structures of this area are complex (fig. 8). The two major fault zones bound crustal domains that differ in potential field character, the Dolsk fault zone (130 km) and the SW edge of a typical three-layered cratonic crust (300 km). Integrated gravity and magnetic model provides evidence of bivergent nature of the Dolsk fault zone. The Dolsk fault zone bounds to the NE the Wolsztyn-Leszno High. This elevated upper crustal structure is reflected by high gravity and relatively high magnetic values. Farther to the NE the edge of EEC correlates with the rapid change in the character of the magnetic field, accompanied by the outstanding gradient zone. A prominent positive magnetic anomaly with the amplitude of approximately 200 nT located NE of this magnetic lineament has been modelled as lower crustal source showing considerable vertical extent and steeply dipping edges. The calculated relatively high magnetic susceptibility (0.06 SI) suggests a mafic intrusion as the source of this anomaly situated on the slope of the EEC. The result of gravity modelling indicates that a high-density lower crust, located beneath a low-density basin reaching a depth of about 20 km, occurs in the area located between these fault zones. This lower crust may represent a wedge of the EEC crust indenting the Palaeozoic Platform crust (Eastern Avalonia ?). However, the calculated density is higher then density of the EEC lower crust. The high-density lower crustal zone may be interpreted as being composed of mafic material that may have resulted from underplating of mantle material. This process could play an important role in the evolution of the Mid-Polish Trough. In order to achieve the match between the observed and calculated gravity effects it was necessary to assume denser uppermost mantle beneath the TESZ. It is also confirmed by seismic investigations along the P4 profile. It may be speculated that increased density in the uppermost mantle of the study area is caused by metamorphic transformation of mafic rocks to eclogite.
7. STRUKTURA LITOSFERY WZDŁUŻ PROFILI P2 I P4 (PROJEKT POLONAISE'97) – 2D MODELOWANIA TERMICZNO-GRAWIMETRYCZNE
Olga Polechońska, Polish Geological Institute, Warsaw
Marta Wróblewska, Polish Geological Institute, Warsaw
Two-dimensional, termal-density models for two profiles of the project: P2 and P4 were created (Polechońska, Wróblewska, 2004). Results of seismic velocity models were the basement for them. First stage of the research was evaluation of temperature distribution model by finite elements method (using “Ansys” software). As the boundary condition the 8oC temperature at the surface level was taken. The heat flow at the bottom of the model was differentiated: from 15 mW/m2 at the craton area to 40 mW/m2 at the Paleozoic platform area. Effect was optimized by surface heat flow (Karwasiecka, Bruszewska, 1997) and temperature distribution at the 1km level (Karwasiecka, 1994). Using abovementioned results the evaluation of densities of middle and lower crust could be done. The formula of Sobolev, Babeyko (1994) was used for that. In the case of the mantle and low-velocity sediments of upper crust, densities were evaluated by choosing method. A this stage the GM-SYS software were used. Figure presents final results of density models for both profiles. While the density distribution for the P4 profile is coherent with the velocities distribution, it doesn’t for the P2 profile. To get the best fidelity between modeled and measured curves, the relatively increased densities of accretion prism sediments in the central part of profile had to be taken.( Fig. 9)
References:
KARWASIECKA M., BRUSZEWSKA B., 1997- Heat Flow Density in Poland. CAG PGI, project nr 21/98, Warsaw, (unpublished).
KARWASIECKA M., 1994, EUROPROBE – opracowanie cieplnego pola Ziemi na obszarze Polski, Oddział Górnośląski Państwowego Instytutu Geologicznego, CAG PGI, Warsaw (unpublished)
POLECHOŃSKA O., WRÓBLEWSKA M., 2004. Deep lithosphere structure along P2 and P4 profiles. Thermal-gravity models. Poster przedstawiony na ( pdf [pdf] (12.12 MB) )
SOBOLEV S., BABEYKO A. Y., 1994. Modeling of mineralogical compositions, density and elastic wave velocities in anhydrous magmatic rocks. Surveys in Geophysics, 15, s. 515-544
8. EDGE OF THE EAST-EUROPEAN CRATON IN THE NORTHWESTERN AND CENTRAL POLAND IN THE LIGHT OF SEISMIC, MAGNETIC AND GEOTHERMAL DATA
Czesław Królikowski, Polish Geological Institute, Warsaw
In this work the current state of knowledge on contact zone of the East European Craton (EEC) with the Palaeozoic Platform (PP) was briefly given and quite new tectonic pattern of the lower crust in the region under study was suggested. The pattern is based on the analysis of the:
- seismic data of the POLONAISE experiment,
- magnetic survey in the north-western and central Poland,
- geothermal field distribution.
The interpretation of the seismic experiment POLONAISE results indicated the cratonic provenience of the lower crust underlying the PP in the area to SW from the TTL. The TTL would denote the edge of the upper and middle crust only. There are three different areas of the magnetic field. The first area is the extensive negative anomaly. To the NE from it, the positive anomaly called the Western Pomerania anomaly (WPA) extends. These two areas are separated by the gradient zone wich runs from Szczecin through Stargard, Piła, Bydgoszcz to Inowrocław. To the north-east from the WPA, the third area of high anomalies extends. The boundary between the WPA and the high anomalies is the big gradient zone and its axis is well known as the Teisseyre-Tornquist Line (TTL) running from Człuchów to Bydgoszcz and Włocławek. The interpretation of the WPA showed that the depth to magnetic body is about 18 km. It roughly corresponds, according to the seismic data, to the bottom of the upper crust of the low seismic velocity (underneath 6000 m/s). Along the south margin of the WPA and to the south from it, the lower crust loses its magnetisation and its presence reveals only due to the high seismic velocity. The magnetisation of the cratonic lower crust can disappear entirely at the Curie temperature. The geothermal modelling shown that the Curie isotherm in this area is at depth 22 – 25 km, considerably shallower than Moho surface. Conscientious analysis of the magnetic anomalies, crust structure, seismic velocity distribution, vertical velocity gradients and geothermal modellings suggest that we have to do with thrusting the cratonic lower crust under the lower crust of the PP ( fig. 10). The following arguments speak for such a geotectonic pattern:
- disappearance of normal magnetisation of the lower crust in the elevation zone of the Curie isotherm to the south from the WPA,,
- presence of the negative anomaly generated by reversely magnetised lower crust of the PP, which overlay the Curie isotherm,
- markedly higher velocity gradient of the lower crust in the PP than that of the cratonic lower crust, what results from occurrence of the low velocity lower crust of the PP over the high velocity cratonic lower crust,
- ensity difference between the cratonic lower crust and the lower crust of the PP; the former is heavier than the latter and the thrusting the former under the latter is more probable rather than the reverse.
There is no single, well defined edge of the Craton. There are three important boundaries which characterize tectonic complicity of the TESZ in this region. The first one is the well known TTL which has been traced on the basis of the analyzing the magnetic anomalies. It is approximate to the deep crust fracture which was determined as a result of seismic soundings in the region. The second boundary is the SW margin of the WPA. It also is the NE extent of the reversely magnetized lower crust of the PP and the zone of the disappearing the magnetic properties of the cratonic lower crust. The boundary would be of magnetic and tectonic character. And the third boundary means the SW extent of the cratonic lower crust.
9. MID-POLISH TROUGH BASEMENT TECTONIC STRUCTURE BASED ON ANALYSIS OF SEISMIC REFLECTION, GRAVITY AND MAGNETIC DATA
Piotr Krzywiec, Polish Geological Institute, Warsaw;
Stanisław Wybraniec, Polish Geological Institute, Warsaw;
Zdzisław Petecki, Polish Geological Institute, Warsaw
Using dense coverage of industrial seismic reflection data from the central and north-western Poland regional tectonic model of the sub-Zechstein basement of the Mid-Polish Trough (MPT) was constructed. This model was based on a concept of regional decoupled Mesozoic evolution of the MPT connected to the presence of the thick Zechstein salt layer which served as a basin-scale mechanical decoupling level (cf. Krzywiec, 2002a,b, 2004; Krzywiec & Wybraniec, 2003). Due to this regional decoupling Mesozoic extension was mostly restricted to the sub-Zechstein basement and Mesozoic sedimentary cover was characterized by lack of significant normal faulting and by gradual thickness changes of syn-extension deposits. Only locally normal faulting did occur within the Mesozoic cover, and this faulting often triggered evolution of salt structures. Mechanical decoupling played also important role during the Late Cretaceous – Palaeogene inversion of the Mid-Polish Trough. Taking into account [1] location of thickness gradients of the Triassic – Jurassic – Lower Cretaceous deposits, [2] location of salt structures, and [3] morphology of the sub-Zechstein basement tectonic map of inferred sub-Zechstein fault zone responsible for the MPT’s extension / subsidence and its inversion was constructed. Results of seismic data interpretation were compared with various geological maps and with processed gravity and magnetic maps, with high degree of correlation was achieved between regional sub-Zechstein tectonic pattern and other geological and geophysical features (fig. 11). NE boundary of the MPT was generally controlled by the SW edge of the East European Craton, while its SW boundary developed along system of en-echelon fault zones most probably inherited from earlier tectonic phases. These en-echelon fault zones conform very well wit location of the Holy Cross Mts. fault zone known from SE Poland. Using in-house developed numerical methods for processing and visualization of seismic reflection data two deep reflection profiles GB-1 and GB-2 were analysed. It was confirmed that GB-1 profile did not provide virtually any information regarding the sub-Zechstein basement. Reprocessed GB-2 profile clearly imaged lower crustal delamination zone (“crocodile structure”) identified earlier by Petecki (2003) and possibly being an equivalent of similar lower crustal collisional zone known from Germany (cf. e.g. Meissner et al., 2002).
References:
Krzywiec P., 2002, Oświno structure (NW Mid-Polish Trough) - salt diapir or inversion-related compressional structure? Geological Quarterly, 46(3): 337-346.
Krzywiec P., 2002 - Mid-Polish Trough inversion - seismic examples, main mechanisms and its relationship to the Alpine – Carpathian collision. [In]: G. Bertotti, K. Schulmann, S. Cloetingh (ed.), Continental Collision and the Tectonosedimentary Evolution of Forelands. European Geosciences Union Stephan Mueller Special Publication Series, 1: 151-165. ( pdf [pdf] (9.34 MB) )
Krzywiec P., Wybraniec S., 2003, Role of the SW Margin of the East European Craton During the Mid-Polish Trough Mesozoic Development and Inversion – Integration of Seismic and Potential Field Data. Proceedings of the 8th Meeting of the Czech Tectonic Studies Group / 1st Meeting of the Central European Tectonics Group, 24-27.04, Hruba Skala, Geolines – Papers in Earth Sciences, 16: 64-66.
Krzywiec P., 2004, Triassic evolution of the Kłodawa salt structure: basement-controlled salt tectonics within the Mid-Polish Trough (central Poland). Geological Quarterly. 48(2): 123-134. ( pdf [pdf] (651 KB) )
Krzywiec P., 2004, Basin-scale basement control of the evolution of the Mid-Polish Trough. American Association of Petroleum Geologists European Region Conference, Prague, 10-13.10.2004, 87. ( pdf [pdf] (35 KB) )
Meissner R., Thybo H., Abramovitz T., 2002, Interwedging and inversion structures around the Trans-European Suture Zone in the Baltic Sea, a manifestation of compressive tectonic phases. Tectonophysics, 360: 265-280.
Petecki Z., 2003, Lower crustal reflectivity pattern in the Pomeranian Segment of the Trans-European Suture Zone: evidence for Caledonian Collision Zone. Acta Geophysica Polonica, 51(1): 63-72.
Wybraniec. S., 2004. Crust reflectivity of NW Europe on the base of BIRP seismic reflectivity project. Presented during the scientific meeting of PGI Geophysical Department, 12 maja 2004.( pdf [pdf-in Polish] (7.22 MB) )
10. INTERPRETATION OF DEEP SEISMIC DATA – RESULTS OF SEISMIC REFLECTION MODELLING
Kaja Pietsch, Department of Geology, Geophysics and Environmental Protection, Academy of Mining & Metallurgy, Kraków;
Marcin Kobylarski, Department of Geology, Geophysics and Environmental Protection, Academy of Mining & Metallurgy, Kraków
The paper presents application sample of 2D seismic modeling for interpretation wavefields recorded during deep seismic (GBS) along GB-2 profile, conducted by PBG Geophysical Exploration Company (1992) and along TO740198 profile, conducted by Geofizyka Toruń Sp. z o.o. (1998). Discussed profiles cross Variscan deformations of south-western Poland, covered by Fore-Sudetic Monocline. Apart from reflections corresponded to sedimentary cover (A), highly reflective zone is visible (C), which consists of subhorizontal series of reflections and is corresponded to lower crust. Transparent zone corresponded to upper crust (B), consisted of individual, low frequency reflections is also visible. The Moho discontinuity occurs at the base of lower crust and was identificated along profiles. Poor quality recordings obtained along GB-2 and TO740198 profiles implies, that geophysical and geological interpretation should be treated as hypothetical (Cwojdziński et al., 1996). In this situation essential tool that assists in interpretation is seismic modeling, that permits constructing of theoretical wavefields, calculated form more and more complicated seismogeological models of investigated structure, to obtain accordance with receorded wavefields. Ambigous results of analized seismic profiles imply, that depth-velocity model interpreted on the base of DSS Polonaise ‘97 P4 profile (Guterch et al. 1997) was used as a starting seismogeological model. Theoretical wavefields were calculated with computer programs based on two different approaches of wave propagation. In first approach, zero-offset wavefield was calculated using finite difference method aproximating solution of full-wave equation. In second approach ray tracing method was used. Modeling was performed using Promax and GeoGraphix sysems (Landmark Graphic Corporation), located at Faculty of Geology, Geophysics and Environmental Protection, AGH Technical University. Calculated wavefields in similar way reflect established seismogeological model, observed seismic boundaries positions resemble positions along GB-2 and TO740198 profiles. Distinct differences are only visible within lower crust (C), where highly reflective zone was recorded, which can be related to laminated structure of this complex (Guterch et al., 1992, 1994). Discrepancy between modeled and recorded wavefields within lower crust indicates on modification necessity of established velocity model. High reflectivity of the complex, suggests existence of laminated structure, with a large velocity contrasts. Velocity modification resulted in change of theoretical wavefield within lower crust that consists of reflects series. Combination of theoretical with recorded wavefields along GB-2 and TO740198 profiles shows conformity in localization of highly reflective zone, that confirms laminated structure of the complex. Performed modeling results in conclusion refered not only to modeling method but also to modeling usfulness for interpretation of deep reflection seismic profiles. Theoretical wavefields calculated with ray tracing method and on the base of finite difference method are similar. Modeled pattern of seismic boundaries is adequate to velocity model, amplitudes are apropriate to velocity distribution within layers. It seems that with frequencies observed at deep seismic reflection experiments and expected precision, ray tracing method is enough efficient. From practical point of view this conclusion is quite essential because for this kind of modeling, velocity structure is simpler and calculation time – shorter. Performed modeling also shows usefulness of seismic reflection modeling for geophysical and geological interpretation of deep seismic reflection profiles. Obtained quite good conformity of poor signal quality wavefields recorded along GB-2 and TO740198 profiles with theoretical wavefields permits assume that final seismogeological model reflects Earth structure within investgated zone. (Fig. 13)
References:
BABEL WORKING GROUP, 1993 – Deep seismic reflection/refraction interpretation of crustal structure along BABEL profiles A and B in the southern Balic Sea. Geophysics J. Int. 112; 325-343.
CWOJDZIŃSKI S., DZIEWIŃSKA L., JÓŹWIAK W., MŁYNARSKI S., POKORSKI J., ZIENTARA P., BAZIUK T., 1996 – Reinterpretacja sejsmicznych badań refleksyjnych na profilach GB-2 i GB-2A. Opracowanie PIG, Warszawa
FEDOROWICZ A., KLECAN A.,1998 – Opracowanie tematu Kościan – Krobia. Archiwum Przedsiębiorstwa Geofizyka Toruń Sp. z o.o.
GUTERCH A., GRAD M., MATERZOK R., PERCHUĆ E., JANIK T., GACZYŃSKI E., DOAN T.T., BIAŁEK T., GADOMSKI D., MŁYNARSKI S., TOPORKIEWICZ S., 1992 – Laminated structure of the lower crust in the fore-Sudetic region in Poland derived from seismic data. Physics of the Earth and Planetary Interiors, 69: 217-223.
GUTERCH A., GRAD M., JANIK T., MATERZOK R., LUOSTO U., YLINIEMI J., LÜCK E., SCHULTZE A., FRÖSTE K., 1994 – Crustal structure of the transition zone between Precambrian and Variscan Europe from new seismic data along LT-7 profile (NW Poland and eastern Germany). Geophysics, C. R. Acad. Sc., 319 (II): 1489-1496.
GUTERCH A., GRAD M., THYBO H., KELLER G.R., 1997 – Polonaise’97 International seismic experiment. Terra Nostra, II: 56-59. KASINA Z., 2003 – Modelowania sejsmiczne. Wydawnictwo GSMiE PAN, Kraków.
MEISSNER R., KRAWCZYŃ C.M., 1999 – Caledonian and Proterozoic terrane accretionin the Southwest Balic Sea. Tectonophysics 314: 123-143.
MŁYNARSKI S., POKORSKI J., DZIEWIŃSKA L., JÓŹWIAK W., ZIENTARA P., 2000 – Deep reflection seismic experiments in western Poland. Geological Quaterly, 44 (2): 175-181.
PETECKI Z., 2003 – Lower crustal reflectivity pattern in the Pomeranian Segment of the Trans-European Suture Zone: evidence for Caledonian Collision Zone. Acta Geophysica Polonica, 51 (1): 63-72.
11. ONE-DIMENSIONAL RHEOLOGICAL LITHOSPHERIC MODELS ALONG THE POLONAISE’97 PROJECT, TTZ AND LT DEEP SEISMIC PROFILES
Marek Jarosiński, Polish Geological Institute, Warsaw;
Marcin Dąbrowski, Institute of Geophysics, Polish Academy of Sciences, Warsaw
Complex crustal structure and significant lateral changes in surface heat flow make the TESZ an interesting object for study of the rheological differentiation of lithosphere. Two step, 1D thermal and mechanical modelling was performed for 5 POLONAISE, TTZ and LT-7 deep seismic profiles. The results of thermal modelling showed that heat production in the EEC type of the crust is significantly less (by ca. 60%) than in the rest of the crust within the TESZ. It points to the hypothetical limit of the Gondvana-derived terranes and the Baltica-type crustal blocks. Based on the seismic velocity structure, which was determined by DDS profiling, petrological models for discriminated lithospheric layers were built. Parameters of the “power law” creep function for given layer were calculated by averaging adequate parameters of constituent petrological types. Rheological modelling for a scope of the most probable strain rates and tectonic regimes lead, in result, to identification of the brittle and ductile stratification of the lithosphere and location of potential mechanical decoupling levels. Also integrated strength of the lithosphere was estimated with differentiation between the crustal and the mantle strength. Consistency of rheological model was compared to the concept of tectonic evolution of the modelled area and possibility of some alternative solutions of rheological model were discussed. (Fig. 14)
11. DEEP BASEMENT STRUCTURE OF THE PALAEOZOIC PLATFORM IN SW POLAND IN THE LIGHT OF SEISMIC RESULTS OBTAINED FROM THE POLONAISE’97 EXPERIMENT.
Stanisław Mazur, Institute of Geological Sciences, Wrocław University, Wrocław;
Marek Jarosiński, Polish Geological Institute, Warsaw
Seismic profiles of the POLONAISE’97 experiment provide evidence for the deep basement structure of the TESZ area representing a broad and complex zone of terrane accretion which separates the old Proterozoic lithosphere of the East European Craton (EEC) from the younger Palaeozoic lithosphere of Western Europe. The obtained results point to essential differences in the seismic structure of a lithosphere characteristic of the EEC, Palaeozoic platform of SW Poland and Variscan orogen. Results of deep seismic sounding combined with potential field and thermal data allows the differentiation of 5 varieties of lithosphere: (1) EEC variety – a lithosphere of the East European Craton showing the three-layer seismic structure of a crust and relatively low seismic velocities in an upper mantle; it is characterized by the low radiogenic heat production and the high amplitude of magnetic anomalies, (2) TTZ variety – lithospheric blocks adjacent to the EEC and extending to the SW margin of the Teisseyre-Tornquist Zone (TTZ); they display the three-layer seismic structure of a crust, the thick low-velocity upper crust and Vp in the upper mantle higher than that below the EEC; the TTZ lithosphere is also characterized by the low production of radiogenic heat (similar to the EEC) and the medium amplitude of magnetic anomalies in the Pomeranian segment, (3) TESZ variety – a lithosphere of the Trans European Suture Zone (TESZ) between the TTZ and the Dolsk Fault Zone (DFZ) which shows the three layer seismic structure of a crust, the thick low-velocity upper crust and the strongly reflective lower crust with a high Vp gradient; the TESZ lithosphere is characterized by the high production of radiogenic heat and the lack of magnetic anomalies, (4) PP variety – a segment of the lithosphere between the DFZ and the Middle Odra Fault Zone (MOFZ) characterized by the two-layer seismic structure of a crust and low seismic velocities down to the Moho discontinuity; similarly to the TESZ variety, the PP lithosphere demonstrates the high radiogenic heat production and the lack of magnetic anomalies, (5) VP variety occurring to the SW of the MOFZ reveals features similar to those typical of the PP lithosphere but remains distinct because of low Vp in the upper mantle; a shallow subcrop of the crystalline basement in that area results in the occurrence of apparent magnetic anomalies. The two former types of a lithosphere (EEC and TTZ) are genetically linked to the EEC whereas the two latter varieties (PP and VP) represent a lithosphere of the Variscan orogen. A lithosphere of the Palaeozoic platform (TESZ variety), embraced between the Variscan orogen and the EEC, represents a separate basement block derived from Avalonia or an Avalonia-related terrane and accreted to the Baltica margin during the Early Palaeozoic. The three-layer seismic structure of a crust in that area is probably produced by a number of superimposed effects like underplatting by magmas melted off from a subducted plate of the Tornquist ocean, thrusting of the Caledonian accretionary wedge and partitioning by multiple younger strike-slip displacements. The results of the POLONAISE’97 experiment verify the role of the Dolsk Fault Zone as the NE boundary of the area affected by a thick-skinned Variscan tectonics. The Variscan-related modification of a seismic structure has its effect not only on a lithosphere of the Bohemian Massif, usually correlated with the Armorica terrane assemblage, but also on a fragment of the Palaeozoic platform of SW Poland located between the DFZ and MOFZ. (Fig. 15)
13. GEOLOGICAL INTERPRETATION OF DEEP SEISMIC REFRACTION DATA OF THE POLONAISE’97 PROJECT – PRECAMBRIAN PLATFORM
Zbigniew Cymerman, Polish Geological Institute, Wrocław
Geological interpretative cross-sections of four seismic profiles (P2, P3, P4 and P5) of the experiment POLONAISE’97 from the Polish part of the East-European Platform (EEP) based upon borehole information and regional geological considerations is presented. The Precambrian crystalline basement in NE Poland has been penetrated by 259 deep boreholes and was located at depths varying from hundreds of meters to a few kilometers. Unfortunately, these boreholes are not uniformly distributed throughout the experiment POLONAISE’97 area, most were concentrated in the Suwałki anorthosite massif and in the Podlasie region. There are problems in the reliability of fabric (SM foliation) orientations using curving axis of boreholes and the nature of the protoliths when we to have no modern geochemistry and little reliable age dating. The results of the structural analysis of the Polish part of the EEP show that the ductile shear zones have developed at a wide range of scales from regionally km thick belts down to mm-thin layers. In general, the dip angle of the SM foliation is about 50o-60o. In the light of structural researches of the drill cores, there is little structural or kinematic data to support previous models of the structure and evolution of the EEP with several old “massifs and younger “orogenic belts”. The tectonic evolution of the Precambrian crust from NE Poland is characterized by two main processes. Older deformations are related strictly with compressional regime and are connected with the development of ductile thrust packages displaced generally towards the SW during amphibolite and granulite facies of regional metamorphism during the Svecofennian orogeny. The next deformations occurred during the Gothian orogeny (ca. 1.55-1,45 Ga), and was characterized by compressional to locally extensional regimes of strain with the development of ductile thrust sheets, some of them are similar to a pop-up geometry (antiformal stack), as e.g. in the SW part of the Suwałki Anorthosite Massif. Overthrusting of the thrust packages was directed in approximately NE-SW direction, but with ductile displacement approximately towards the NE. The development of rapakivi type granitic magmatism of the Masurian complex was also connected with this younger orogeny. (Fig. 16)
Designed & Webmastered by: Marta Wróblewska19 th November 2004
Note: Some of the contact information may not be valid.